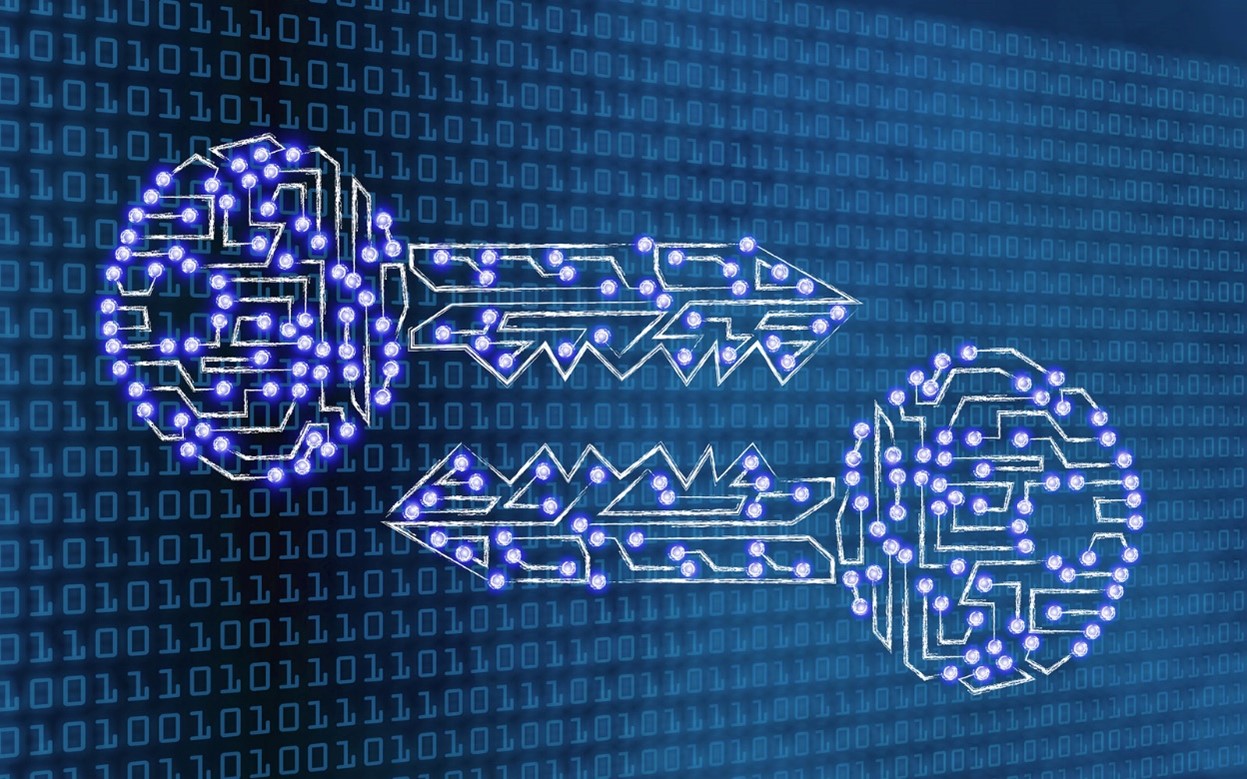
What is encryption?
The purpose of cryptography is to transform a message into a version that can’t be understood, and can only be read by someone who has the ‘key’ or method to revert the scrambled message to its original state. The idea is to send encrypted messages that can only be read by the intended reader who has the correct ‘key’.
However, many methods of encoding messages can be decoded by people other than the intended reader. For example, methods of cryptography based on wordplay, or by mathematical codes, can be decrypted by reversing that process.
What is quantum encryption?
Put simply, the aim of quantum encryption is to use the basic principles of quantum mechanics to encode a message in such a way that it would be literally impossible to decrypt without already being given the method—in other words, impossible to decrypt by anyone other than the intended reader.
How does quantum encryption work?
The most common theory of quantum encryption is known as quantum key encryption, and makes use of photons (particles of light) being in a quantum state. This is a state between real states (ways we would normally think of particles existing), where a particle will assume one of these real states after being observed.
To send a message using this method of encryption, the sender transmits photons through a filter, which will ensure they are in one of a certain number of real states. This can be thought of as sending a signal down a fibre optic cable and passing it through a polarising filter.
The receiver passes the photons through a series of filters, which indicates which real state each photon is in. The receiver and sender then compare the states of each photon sent and photon received, and the transitions between states form a pattern—or key—that can be used to interpret future messages.
Because a particle in a quantum state will always become a real state when observed, then if the photon message arrives in a pattern which does not match the key, the receiver knows that the message has already been seen. In other words, if any part of the message has already been intercepted or seen—down to one particle—then the receiver and sender will immediately know, and can change their code.
What are the drawbacks of quantum encryption?
Despite its apparently impervious design, there are a couple of reasons why quantum encryption is not in widespread use. The first is expense: quantum encryption still relies on very new technology, and it would therefore take a lot of time and money to transmit even a single message using this method.
Another issue is that while the quantum encryption part of sending a message is theoretically impossible to hack, any messaging system will also require non-quantum elements which will still be vulnerable to hacking. While quantum key encryption is the most promising method of encryption for the future, the technology required to make it practical in commonplace use is not yet there.
Hopes for the future
As mentioned above, one of the current main limitations on the use of this technology is the large and expensive technology required to implement it. One component of this technology which has seen significant progress is the Superconducting Quantum Interference Device (SQUID), of which there are several examples in the Science Museum Group Collection.
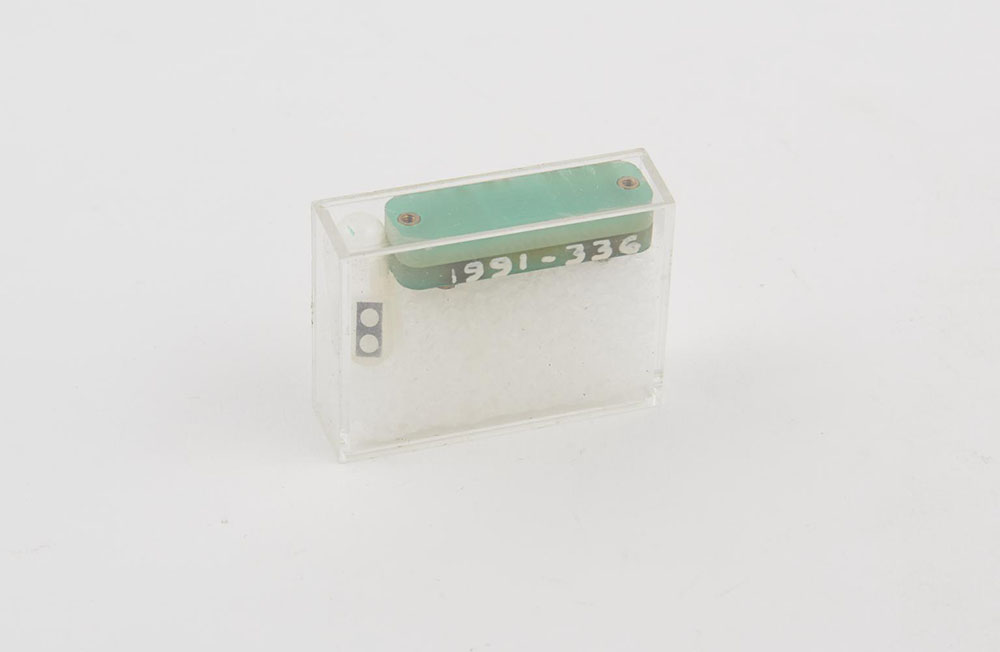
These components sense tiny changes in an electric or magnetic field, and are currently most commonly used in medical technology for detailed scans of patients. Because of their sensitivity, they will only operate at very low temperatures, requiring specific conditions to manufacture and operate them.
Three variations of these SQUIDs are currently on display at the National Science and Media Museum as part of our exhibition Top Secret: From Ciphers to Cyber Security. Because of limitations like the expense and complexity of quantum technology like these SQUIDs, the application of cryptography methods like quantum encryption is currently reserved only for the high security branches of government and banks which can afford the experimental new technology required.
Interested? Learn more about cryptography at Top Secret: From Ciphers to Cyber Security—the exhibition is at the National Science and Media Museum from 11 February to 5 June 2022.